MEDIA RELEASE
17 May 2022
Supporting national science ambition with giant computing grants
NCI Australia is pleased to announce the recipients of the 2022 Australasian Leadership Computing Grants (ALCG) supported by the Australian Government through the National Collaborative Research Infrastructure Strategy (NCRIS).
The three successful projects cover a search for new kinds of insecticides, fundamental investigations of electron-molecule collisions and next-generation low-emissions electricity technology.
The researchers will use substantial grants of computing time to work on some of the most complex problems facing science today. The highly-competitive ALCG process calls for research groups to propose ambitious and innovative projects beyond the scale of typical computational workloads.
NCI Director Professor Sean Smith says that the successful ALCG recipients show what great science and great computational expertise can be. He says that the successful projects reflect the world-class, high-impact research that NCI enables on its nation-leading Gadi supercomputer.
“The researchers selected through this scheme are pushing the envelope in bringing computational resources to bear on some of our most pressing issues. The high-quality applications received in this round showcase the growing depth and breadth of compute-driven investigations and are a testament to the tremendous scientific talent in Australia,” said Professor Smith.
Three projects have been awarded almost 140 million units of computing time, which is equivalent to almost 20,000 years of constant calculations on one single computer.
Find out more about the Australasian Leadership Computing Grants here.
_______________________________________________________________
The three recipients of the 2022 Australasian Leadership Computing Grants are:
Dr Evelyne Deplazes, The University of Queensland
Dr Deplazes will be running detailed simulations of a naturally occuring toxin, a promising candidate for developing into an environmentally friendly bioinsecticide for use in pest control and crop protection.
Professor Igor Bray, Curtin University
Professor Bray will be performing powerful electron-molecule collision simulations and produce a comprehensive dataset of collision data for use in fusion energy and astrophysical plasma science in the future.
Professor Richard Sandberg, University of Melbourne
Professor Sandberg will be conducting world-first simulations of air flow in Hydrogen-powered turbines, for use in the development of next-generation hydrogen power plants.
_______________________________________________________________
Research will take place on NCI’s Gadi supercomputer, commissioned in early 2020, and funded under the Australian Government's National Collaborative Research Infrastructure Strategy (NCRIS). NCI Australia brings the Australian Government and the Australian research sector together through a collaboration involving CSIRO, The Australian National University, Bureau of Meteorology, Geoscience Australia, universities, industry and the Australian Research Council.
_______________________________________________________________
Research Project Details
Bacterial Toxins as Environmentally friendly insecticides
Dr Evelyne Deplazes
Dr Michael Landsberg
The University of Queensland
Insecticide resistance poses an increasing threat to global food security, biosecurity and the Australian grain, fruit, and vegetable industry. Pore-forming bacterial toxins are promising candidates for developing more effective bioinsecticides for pest control and crop protection. One of the barriers to converting these naturally occurring toxins into commercial insecticides is a lack of structural models and our limited understanding of the pore-forming mechanism.
YenTc is a bacterial toxin that shows potent insecticidal activities in crop pests resistant to other bioinsecticides. This project will use the unpublished, high-resolution structure of the YenTc pre-pore and pore-forming states to carry out the first atomistic simulations of the full-size YenTc complex.
The simulation in this project pushes the boundary of modelling large protein complexes at the atomistic scale and has the potential to deliver leading-edge structural models and insight into the pore-forming mechanism of bacterial toxins that cannot be achieved with experiments alone or standard simulation approaches. The structural models will provide pre-requisite knowledge for the future development of novel biopesticides. This knowledge will ultimately be essential to attracting co-investment from potential industry partners in the Agritech and Biotech industries.
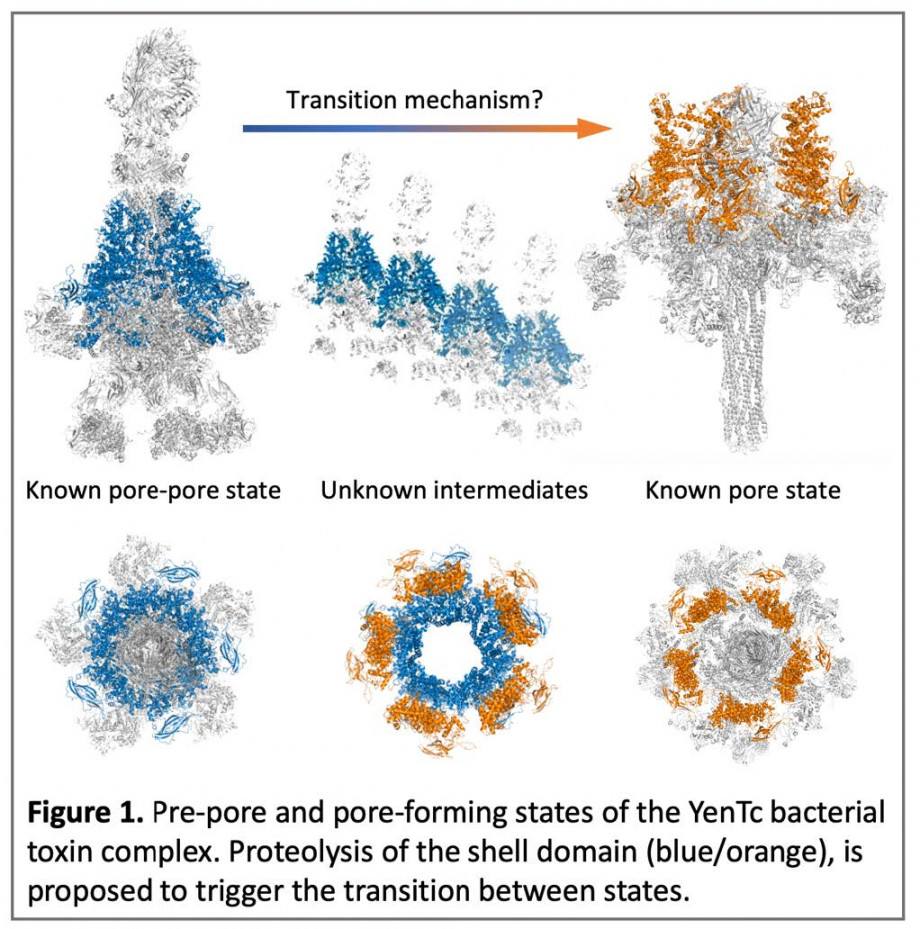
_______________________________________________________________
Electron-molecule collision modelling for fusion and astrophysical applications
Professor Igor Bray
Professor Dmitry Fursa
Dr Liam Scarlett
Curtin University
Collisions involving molecules are ubiquitous throughout the universe. Consequently, there is no shortage of applications that benefit from their quantitative understanding, particularly in the fields of fusion energy and astrophysics. In this project we will apply our uniquely powerful quantum reaction techniques to model electron collisions with the helium, lithium, and beryllium hydride molecules and their ions, and thereby produce comprehensive data sets for application in fusion and astrophysical plasma modelling. The International
Thermonuclear Experimental Reactor (ITER), the largest scientificproject on the planet, is just a few years away from first plasma. Together through continuing collaborations with the International Atomic Energy Agency and the Max-Planck Institute of Plasma Physics we will supply the data which is essential to the understanding of the underlying collision processes. The same collisions are just as important in astrophysics, and with the successful launch of the James Webb telescope, we will provide comprehensive data sets in order to understand the origin of the uniquely detailed upcoming data.
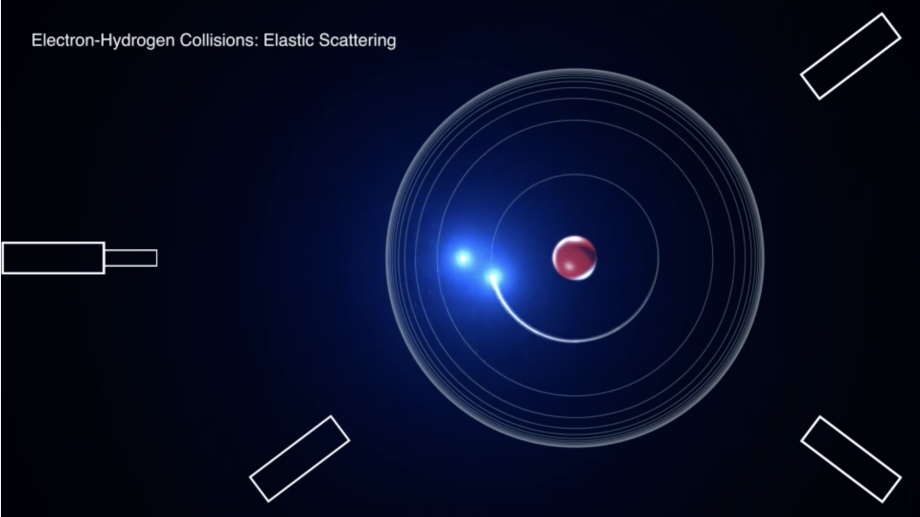
_______________________________________________________________
Hydrogen co-firing for power generation: what happens in the turbine?
Professor Richard Sandberg
Dr Massimiliano Nardini
Dr John Leggett
Dr Melissa Kozul
Dr Pawel Przytarski
University of Melbourne
To support the required energy transition towards net-zero, gas turbines will need to be adapted in order to burn increasing fractions of hydrogen. New combustors targeting hydrogen co-firing, or even pure hydrogen combustion, will undoubtedly produce different inflow velocity and temperature characteristics for the power-producing turbine. The combined effects of velocity and thermal profiles and fluctuations affects the intricate physical mechanisms of the turbine blade boundary layers and can have detrimental effects on blade performance and life span. Furthermore, the potentially increased losses and thermal stresses can be further exacerbated by blade surface roughness, that inevitably occurs in operation, yet is typically neglected in numerical studies. Understanding and predicting the concerted action of inflow conditions indicative of hydrogen co-firing with operational surface roughness of the blades is crucial. In this project, we therefore plan to perform world-first extreme-scale simulations that incorporate both future engine-relevant inflow conditions, along with surface textures representative of in-service blade degradation, to study the detailed flow physics of power-generating turbines. The data produced by this proposal will permit better predictions of power station output with hydrogen co-firing, and furthermore support development of a turbine more efficient for hydrogen co-firing and in long-term service. The world-first simulations will be carried out utilizing the well-validated in-house code HiPSTAR, a high-order compressible flow solver, that has been ported and optimized to fully exploit the GPUs on Gadi. Sophisticated post-processing of the data will be performed to solve several crucial research questions, such as how a representative range of inlet conditions affects laminar-turbulent transition of the turbine boundary layers, in particular when the turbine surface is rough due to expected in-service degradation. The adoption of the new knowledge and models derived from the high-fidelity data by our industry partners will support hydrogen uptake and ensure efficiency gains in future engines with economic and environmental benefits.
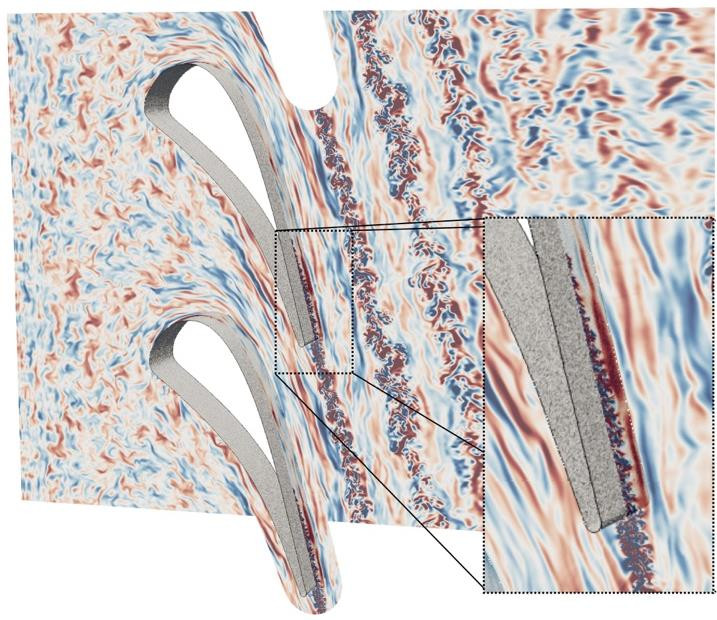
_______________________________________________________________
Media enquiries: NCI Communications and Outreach Manager – Adam Huttner-Koros, adam.huttner-koros [@] anu.edu.au